Research Overview
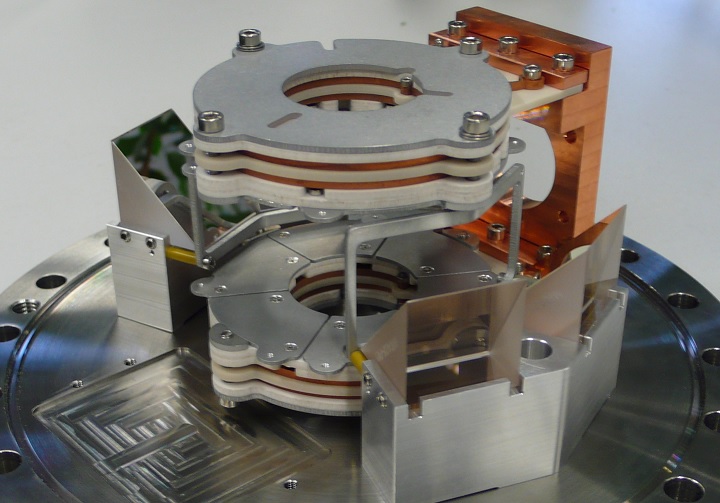
My main research focus has been the investigation of cold and ultracold ensembles of polyatomic molecules. Molecules at very low temperatures allow fundamental experiments of quantum physics at the molecular, atomic, and sub-atomic level. As a first example, precision spectroscopy of low temperature molecules allows to test the foundations of physics. This includes searching for physics beyond the standard model of particle physics and probing for dark matter. Low temperature molecules also allow to perform cold and ultracold collision and chemistry experiments. Such experiments help understand chemical processes at a quantum level. A third area of application involves the use of molecules to investigate quantum many body systems. Cooling molecules to near absolute zero allows to investigate new quantum phases of matter such dipole bound quantum droplets. Finally, molecules are promising building blocks for realizing a quantum computer, possibly allowing to harness quantum physics to realize unimaginable computing power.
For many years, my cold and ultracold molecule research primarily involved developing suitable techniques to generate ensembles of molecules at very low temperatures. I have been the principle investigator developing the optoelectric cooling method for molecules. This Sisyphus-based cooling approach is the most widely applicable technique for dissipatively cooling molecules to very low temperature, and has allowed us to cool formaldehyde (H2CO) molecules to the ultracold (sub-mK) temperature regime. Moreover, I have been involved in the cryofuge experiment, combining buffer-gas cooling with centrifuge deceleration to generate dense, cold, and slow beams of polar molecules. Recently, we have loaded these molecules in a microstructured electric trap, allowing to investigate cold molecule-molecule collisions. A main future aim is to load ultracold molecules in a microwave trap, allowing to realize a quantum degenerate gas of polyatomic molecules.
Based on the success generating low temperature ensembles of polar molecules, we have been increasingly focused on applications of cold and ultracold polar molecules. We have demonstrated high precision mm-wave spectroscopy on formaldehyde molecules cooled to the low mK temperature regime. Moreover, we have performed detailed investigations of electric field dependent collisions between trapped methyl fluoride (CH3F) molecules.
A second main line of research has been the investigation of interactions between polar molecules and Rydberg atoms. This has been motivated by the possibility to efficiently and nondestructively detect polar molecules using Rydberg atoms. However, combining molecules with Rydberg atoms can also be used as a testbed for quantum particle-particle interactions, as well as for applications such as precision spectroscopy, quantum state control, and quantum information processing. First experiments involved studying molecule-Rydberg-atom interactions at room temperature. More recently, I have set up an experiment to study interactions between slow molecules produced via velocity filtering and Rydberg atoms excited from ultracold atoms in a magneto-optical trap.
A final area of research has been the investigation of high order modes of optical resonators. I have developed new mathematical techniques to solve the Maxwell equations in an optical resonator based on spheroidal wave functions. This allowed us to calculate resonator mode splittings beyond the commonly used paraxial approximation. A particular fascinating aspect of this research has been insight into the coupling of polarisation and the orbital angular momentum of light inside a resonator.