Research Details
Contents
Optoelectric cooling of polar molecules
Cooling molecules by replacing photon recoil with electric field interactions
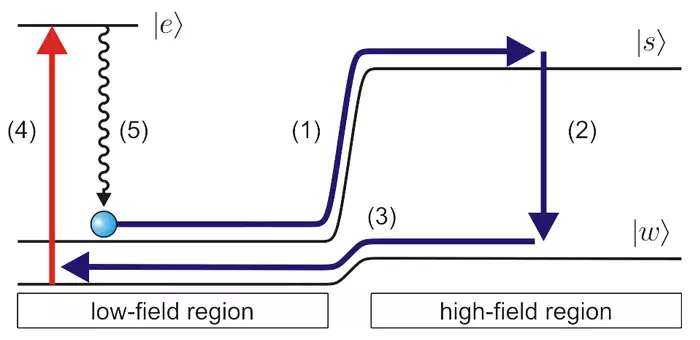
When I started my PhD work on cold molecules in 2006, a number of techniques had been established to produce molecules at temperatures slightly below 1K, including buffer-gas cooling, Stark deceleration, and velocity filtering. However, finding suitable techniques to further reduce the temperature and increase phase-space density was a major open challenge. In particular, standard laser cooling techniques for atoms were believed not to be applicable to molecules at the time.
The key idea which enabled our subsequent work was to replace photon recoil, used in laser cooling to exert a force on atoms, by stronger state-dependent forces via external electric fields. This allows to use radiative spontaneous decay, the key resource to remove entropy in laser cooling, much more efficiently. Specifically, radiation fields manipulating the internal molecular states are applied such that molecules move from low to high electric fields in an electric trap experiencing a strong force towards low electric fields, whereas they return to low electric fields while experiencing a weak force towards low electric fields. The kinetic energy of molecules is thereby reduced, corresponding to a temperature reduction of the molecular ensemble.
M. Zeppenfeld et al., Phys. Rev. A 80, 041401(R) (2009)
A microstructured electric trap: confining cold molecules for over a minute
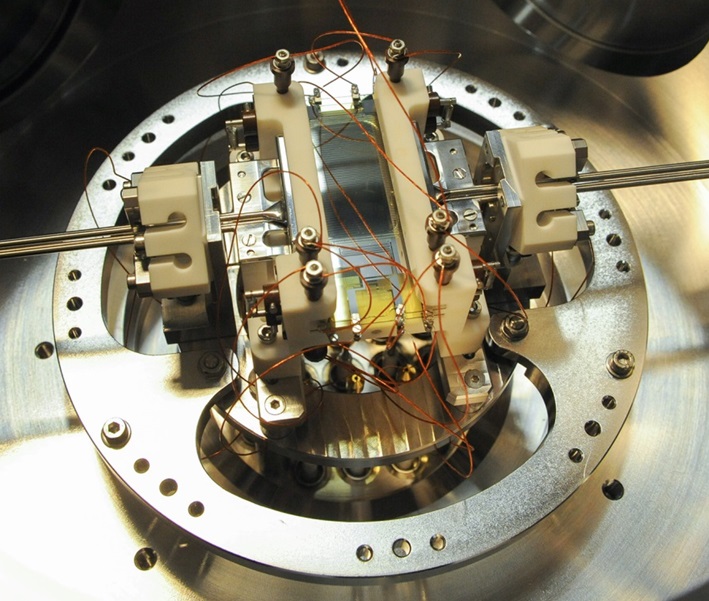
An essential component for realizing optoelectric Sisyphus cooling of molecules, as well as other advanced experiments with cold molecules, is a suitable trap for confining molecules in space while experiments are performed. For this purpose, we designed a unique electric trap, based on microstructured electrodes. Specifically, a pair of glass plates is covered with a periodic sequence of narrow metal strips. With alternating dc voltages applied to neighboring strips, high electric fields are created near the plate surface, repelling molecules in suitable rotational states. Away from the plate surface, the field amplitude rapidly decreases. As a result, placing the two plates face-to-face in a capacitor plate configuration allows to confine molecules in one dimension in a predominantly homogeneous low-field environment. An additional macroscopic electrode around the capacitor plate perimeter allows for full three dimensional confinement of molecules.
Our trap design has delivered remarkable performance. Even for molecules directly loaded from a velocity filtered source we have achieved trap lifetimes of over 10 seconds, with trapped molecules still observed after over a minute of trapping. In later experiments, cooling of molecules increased the trap lifetime to almost a minute. The ability to trap molecules in predominantly homogeneous fields has been invaluable for precise manipulation of molecular states.
B.G.U. Englert et al., Phys. Rev. Lett. 107, 263003 (2011)
Optoelectric cooling of methyl fluoride molecules
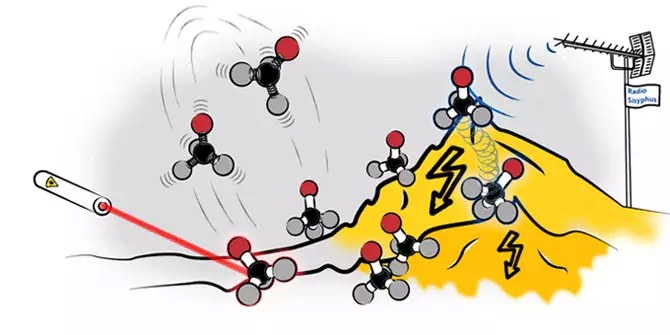
Once molecules are confined in a trap, the essential ingredient to realize optoelectric Sisyphus cooling is to add suitable radiation sources to drive molecular transitions. Driving transitions to an excited state with a reasonably fast spontaneous decay rate is particularly important since the subsequent spontaneous decay allows to reduce entropy, an essential component of any dissipative cooling process. Due to the use of small closed-shell molecules in our experiments, using electronically excited states is not an option since doing so would cause our molecules to fragment. Instead, we excite vibrational modes in our molecules with an optoparametric oscillator at a wavelength of about 3μm. In addition, cooling requires mm-wave radiation to drive transitions between different molecular rotational states as well as microwave radiation to drive transitions between rotational sublevels.
Our initial efforts at cooling were focused on the symmetric top molecule methyl fluoride (CH3F). After considerable optimization, we were able to achieve quite a spectacular result: reducing the temperature of an ensemble of methyl fluoride molecules by a factor of 13 to just below 30mK while increasing the phase-space density by a factor of 30. This was the first demonstration worldwide of laser-based cooling of a trapped molecular ensemble.
M. Zeppenfeld et al., Nature 491, 570 (2012)
Internal state control of polar molecules
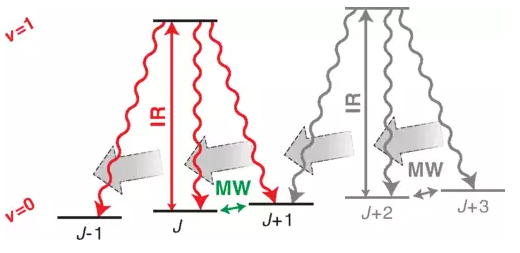
At room temperature, molecules populate thousands, millions, or even more internal rotational and vibrational states, depending on the size of the molecule. In contrast, for quantum experiments, a pure population in only a single quantum state is often desired. While our initial method to produce cold molecules, velocity filtering, reduces the number of populated states by only providing molecules in low-field-seeking states, individual quantum states are nonetheless populated by only a small fraction of molecules. Optoelectric cooling substantially increases the state purity, with molecules predominantly populating two rotational sublevels at the end of the cooling process. However, this increase in state purity to a large degree results from throwing the away molecules initially not in the states used for cooling. Moreover, even having molecules spread over only two sublevels is often insufficient or a major disadvantage for quantum experiments.
As a major improvement to our experiments, we have demonstrated internal state cooling of polar molecules via optical pumping on a vibrational transition. By coupling a number of decay channels of a given excited state to the excited state, molecules are transferred to those decay channels which are left uncoupled. Leaving only a single decay channel uncoupled will eventually transfer the entire population of molecules to the single state. Based on this principle, we were able to incorporate additional rotational states into the optoelectric cooling scheme, thereby increasing the number of cooled molecules. Moreover, once cooling has taken place, we were able to demonstrate a transfer of at least 70% of all trapped molecules to a single rotational sublevel. In subsequent research, this was further improved to over 80% of molecules in a single rotational sublevel.
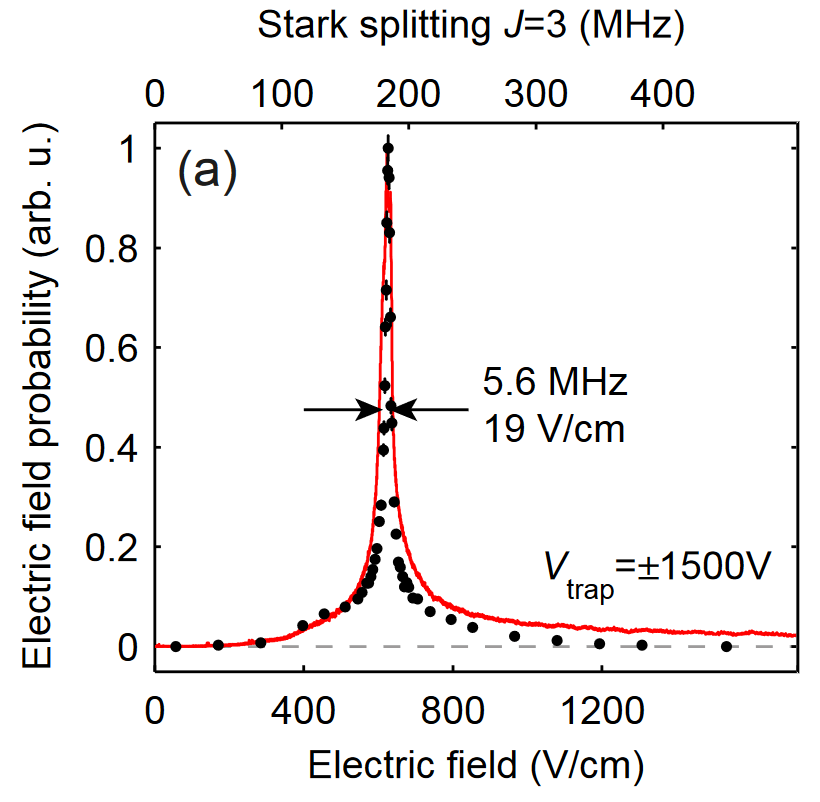
An integral part of our research on internal state control was to develop methods to measure the population in different rotational states. This was necessary since our method to detect molecules using a commercial quadrupole mass spectrometer is state insensitive. State sensitive detection is achieved by eliminating the population from a given set of states in the trap by driving transitions from this set of states to untrapped states. The reduction in signal corresponds to the initial population in this set of states. By adding or subtracting individual states to the set of states which is removed allows to infer the population in indiviual rotational sublevels. However, a major challenge for this research is to sufficiently isolate transitions between various pairs of molecular states such that, e.g., the further reduction in signal when eliminating one state more can be attributed entirely to the additional state.
An ancillary benefit of developing rotational state detection techniques has been the ability to map the electric field distributions inside our trap. Specifically, probing the rate with which a given molecular transition is driven for various detuning caused by the molecular Stark shift indicates the relative probability for a molecule to reside at a given electric field strength. The resulting narrowly peaked distributions vividly illustrate the homogeneous electric fields inside our electric trap.
R. Glöckner et al., Phys. Rev. Lett. 115, 233001 (2015)
R. Glöckner et al., New J. Phys. 17, 055022 (2015)
Formaldehyde cooled to sub-mK temperatures
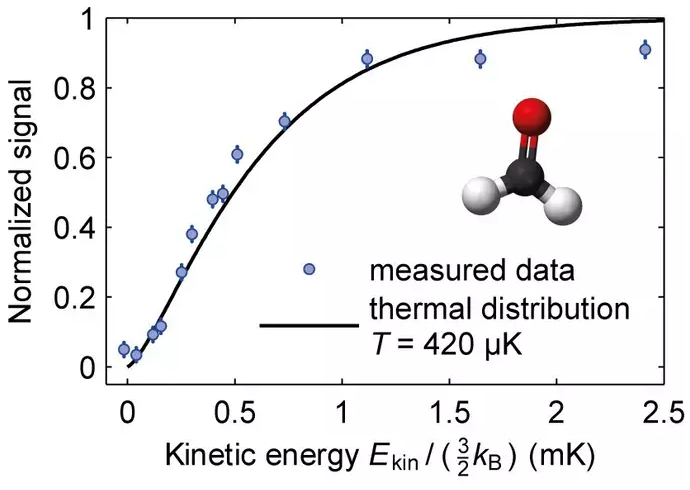
While a variety of direct cooling methods for molecules relatively easily reach sub-Kelvin temperatures, reaching the ultracold temperature regime, roughly corresponding to sub-millikelvin temperatures, for many years seemed out of reach. While our results on optoelectric cooling of methyl fluoride pointed in the right direction, further progress with this molecule was severely limit by the slow ∼15Hz spontaneous decay rate of the excited vibrational state used for cooling. The major breakthrough was achieved by switching to the molecule formaldehyde (H2CO) with a four times larger spontaneous decay rate. Resolving additional challenges such as optimizing 20 cooling steps, reducing the trap potential as cooling progresses, and avoiding inversion transitions, allowed us to break the millikelvin barrier, and achieve temperatures as low as 420μK. Such low temperatures allow to access a new regime for quantum collisions and chemistry, and allow to load molecules into shallow microwave or optical dipole traps which provide major advantages for quantum experiments. Our results represented the first direct dissipative cooling of a gas of molecules to sub-millikelvin temperatures of any experiment worldwide, in parallel with the group of Dave DeMille.
A. Prehn et al., Phys. Rev. Lett. 116, 063005 (2016)
The Cryofuge: centrifuge deceleration of buffer-gas cooled molecules
A centrifuge for polar molecules
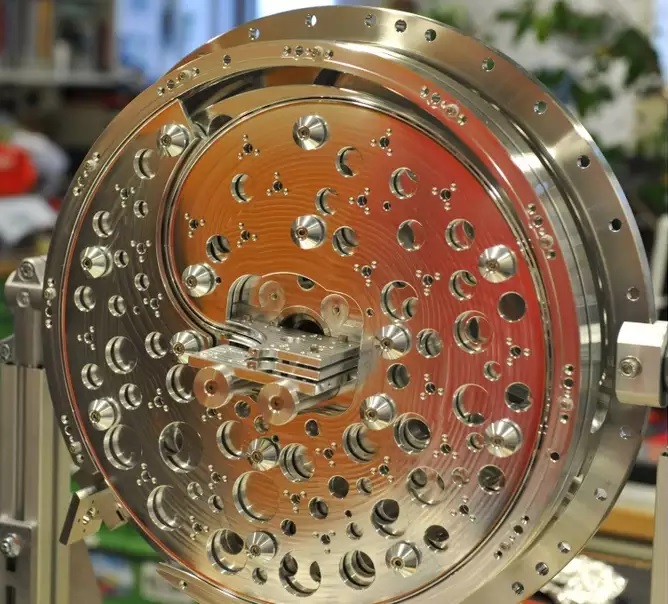
A fundamental disadvantage of the initial source of molecules used for the optoelectric Sisyphus cooling experiments, velocity filtering, is that it provides no initial cooling of the internal molecular degrees of freedom. This limits quantum applications to light molecules, for which the number of internal states populated at room temperature is manageable. To circumvent this problem, we have explored an alternative technique to produce cold molecules, specifically cryogenic helium buffer-gas cooling. However, while buffer-gas cooling can dramatically reduce the temperature of the internal molecular degrees of freedom, it introduces a different problem. The minimum helium density required to provide a sufficient number of helium-molecule collisions to allow for collisional cooling automatically accelerates the molecules when extracting a molecular beam from the buffer-gas cell. In particular, this eliminates the slow molecules which might be subsequently loaded into, e.g., an electric trap. Loading molecules extracted from a buffer-gas source into a trap thus requires deceleration of the molecular beam.
Since our initial molecule sources provide continuous molecular beams and our electric trap is able to load a beam of molecules continuously, a deceleration technique which also operates continuously is strongly desired. One option would be to use gravity. However, reducing the velocity by the roughly 100m/s which is required would require an experiment with a vertical height of 500 meters. To keep the size of the experiment manageable, we instead effectively enhance gravity using the centrifugal potential on a rotating disk. Molecules in a lab fixed quadrupole electric guide are transferred to an electric guide mounted on a rotating disk. Here, fast molecules are forced to climb the centrifugal potential towards the center of the disk, resulting in deceleration, and are extracted along the axis. A clever design of the transfer from the lab-fixed to the disk mounted guide at the edge of the disk allows for a continuous transfer of molecules. The performance of the centrifuge has been quite extraordinary, and allows to decelerate molecules with a velocity up to about 200m/s.
S. Chervenkov et al., Phys. Rev. Lett. 112, 013001 (2014)
Thermometry of buffer-gas cooled molecules
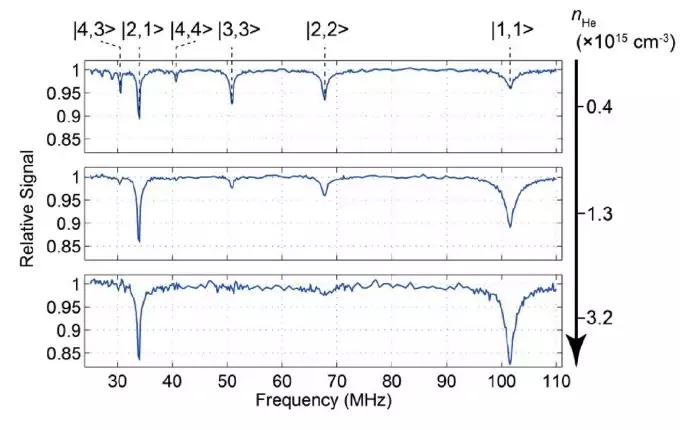
Before combining our buffer-gas source with the centrifuge, an open question was to experimentally verify the degree to which the buffer-gas cell provides for internal cooling of the molecules. For this purpose we installed a pair of capacitor plates in our experiment, interrupting the quadrupole guide over a length of about 2cm. Due to homogeneous electric fields between the capacitor plates, applying radiofrequency radiation to molecules traversing the capacitor plates allows to state selectively drive molecular transitions, leading to loss of molecules in selectable rotational states. Combined with detailed trajectory simulations from the exit of the buffer-gas cell, via the quadrupole guide, through the capacitor plates, and to the quadrupole mass spectrometer used for molecule detection, this allows to infer the molecule state distribution at the exit of the buffer-gas cell.
Using the state detection setup allows a detailed investigation of the effect of buffer-gas cooling on the molecules. Varying the density of the helium buffer gas in a lower density regime allows the transition from little to strong thermalization of the rotational temperature of the molecules with the cell temperature to be observed, resulting in a rotational temperature down to about 6K. For sufficient helium density, the rotational temperature of molecules closely tracks the cell temperature as the cell temperature is varied by roughly a factor of two. Finally, operating the cell in a higher density regime with a neon buffer gas at a cell temperature of 18K results in a rotational temperature of the molecules down to about 2K, far below the cell temperature, due to adiabatic expansion of the higher density gas at the cell exit. We also use the setup to determine the velocity distributions of the molecules at the buffer-gas cell exit, providing further insight into the dynamics of buffer-gas cooling.
X. Wu et al., ChemPhysChem 17, 3631 (2016)
A cryofuge for cold collision experiments
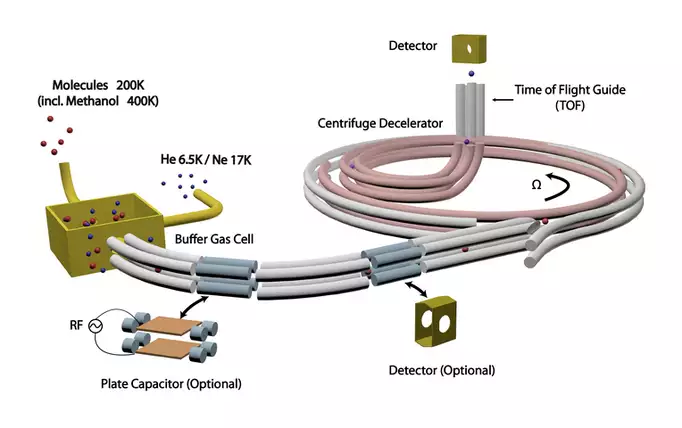
For simplicity, initial testing of our centrifuge molecule decelerator was performed with a velocity filter source of cold molecules, and the full combination with the cryogenic buffer-gas cell was realized as a second step. Combining the two experiments provides a source of molecules which combines multiple desirable features, specifically to create ensembles of molecules in a molecule-species independent manner which are cold, dense, and slow. Cold means both a low relative velocity between individual molecules and a low internal temperature, resulting in lower inhomogeneous broadening and higher state purity for performing quantum experiments. Higher density means a larger number of molecules for a given sample volume, and is particularly relevant to allow collisions between molecules to be investigated. Finally, slow means a low absolute velocity in the laboratory frame, enabling long observation times of the molecules, not least by allowing molecules to be trapped.
The low velocity and high density of molecules exiting the cryofuge directly enable observation of cold molecule-molecule collisions. Both elastic collisions which allow molecules to overcome the transverse confinement in the quadrupole guide as well as inelastic collisions result in a reduction in the number of molecules arriving at the detector. Here, the key signature indicating collisional loss is a telltale dependence on density and molecule velocity. Specifically, the time for molecules to travel to the detector is inversely proportional to the molecule velocity, and slow molecules are thus more likely to collide. Accordingly, we observe a density dependent reduction in the proportion of slow molecules arriving at the detector. Observing molecule-molecule collisions already with the short interaction time provided by time of flight from the centrifuge exit to the detector promises great potential for systematic collision studies once the molecules are loaded into an electric trap.
X. Wu et al., Science 358, 645 (2017)
Simulating cooling dynamics in a helium buffer-gas cell
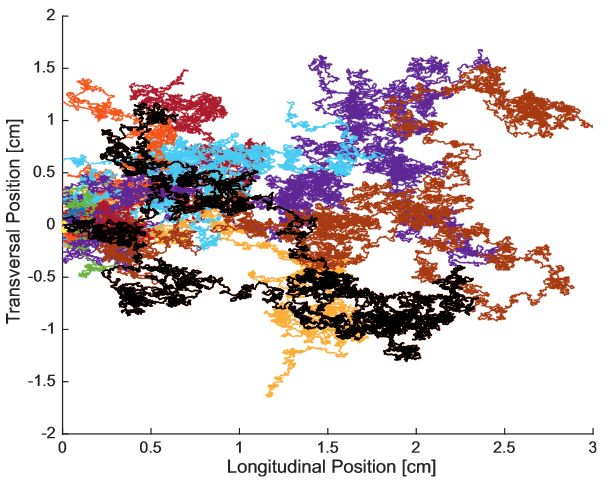
To improve the performance of our cryogenic buffer-gas cell, we have performed simulations of the cell dynamics. Specifically, we use a simple model for elastic collisions between molecules and helium atoms and assume a spatially homogeneous thermal distribution of helium atoms inside the cell (with slight modifications near the cell exit). We calculate individual molecule trajectories starting at the cell molecule inlet, with molecules performing a random walk inside the cell due to collisions with helium atoms. This allows to simulate the properties and number of molecules reaching the cell exit for various cell parameters. Our model allows to reproduce key features observed in the experiment, including thermalization of molecules with the helium buffer gas, a strong reduction in molecule signal for helium density exceeding the minimum required for thermalization, and the loss of slow molecules at the cell exit due to the boosting effect. The simulations provide various insight for improving cell performance.
T. Gantner et al., J. Phys. B 53, 145302 (2020)
Microwave trapping of ultracold molecules
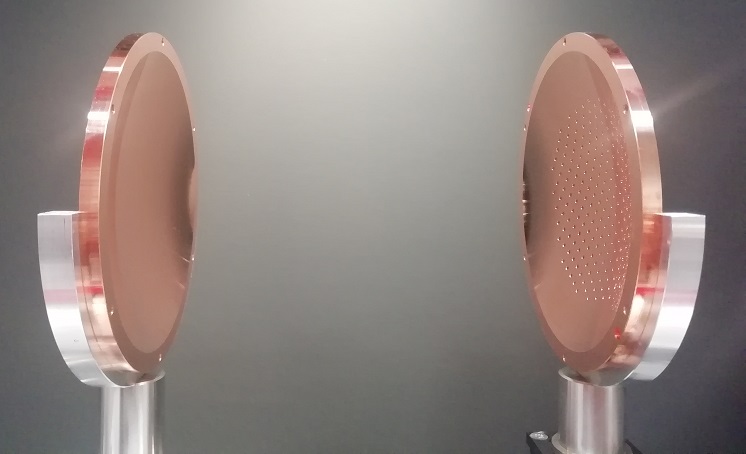
While optoelectric cooling of molecules in our microstructured electric trap has allowed us to reach sub-millikelvin temperatures, further progress towards a quantum degenerate molecular gas as well as towards other quantum experiments with molecules would be greatly facilitated by transferring molecules to a different trapping environment. One option is to load our sub-millikelvin molecules into a microwave trap, with intense microwave fields inside a microwave resonator providing a trapping potential for molecules. A microwave trap would provide substantial benefits including greatly improved optical access, the ability to trap the absolute molecular ground state, improved electric field control, and a more localized ensemble of molecules.
As a first step, we have designed and built a suitable microwave resonator for generating the required intense microwave fields. We realize an open resonator, based on a pair of curved mirrors, thereby providing for excellent optical access to the trapped molecules ensemble. Microwave radiation is coupled into the resonator through a regular series of holes drilled into one of the two mirrors. The precise hole profile is based on FEM simulations to allow for low loss critical coupling of microwaves into the resonator. We achieve a critically coupled resonator finesse of about 2000 for a microwave frequency of 50GHz. This allows for a trap depth of a few millikelvin for formaldehyde molecules with about 20W of microwave power coupled into the resonator.
Applications of cold and ultracold polar molecules
High precision "magic"-field spectroscopy of formaldehyde
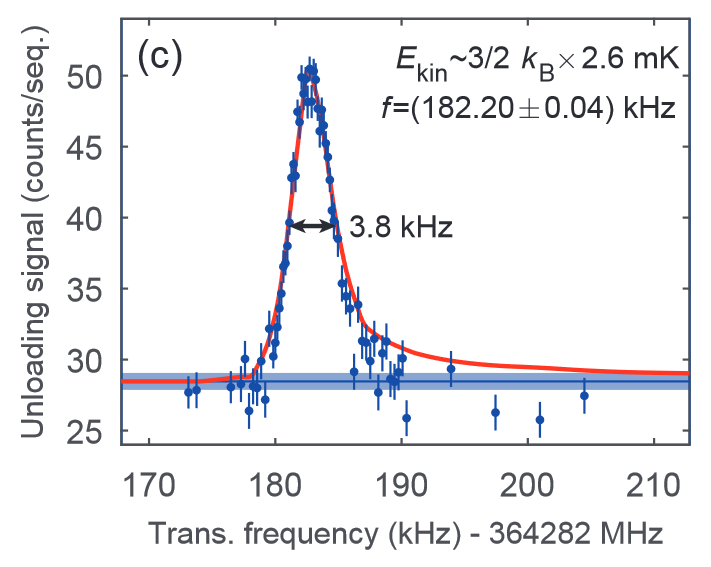
Optoelectric cooling of formaldehyde to the low millikelvin temperature regime combined with our unique microstructured electric trap has allowed us to demonstrate high-precision millimeterwave spectroscopy on a rotational transition of formaldehyde. A major challenge is to circumvent residual Stark broadening which exists despite the homogeneous electric fields inside our trap. For this purpose we apply a "magic" offset field inside the trap at which the slope of the molecule transition frequency with respect to electric field strength is zero for a specific transition. We thereby reduce Stark broadening by roughly 7 orders of magnitude.
We measure Doppler limited linewidths down to 3.8kHz for a transition frequency of about 364GHz, and determine the center line position with a relative precision of about 2×10-10. Our method allows a more precise determination of molecular constants and is a major step towards observing coherent dynamics in trapped polyatomic molecules. Further improvements will enable exciting applications such as measuring parity violation in chiral molecules and placing more stringent limits on time variation of fundamental constants.
A. Prehn et al., Phys. Rev. Lett. 127, 173602 (2021)
Electric-field controlled collisions of trapped methyl fluoride molecules
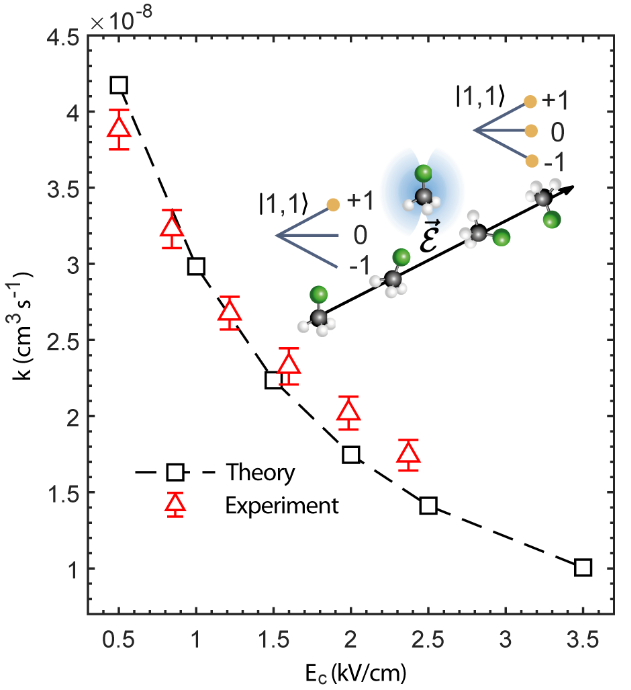
Loading molecules from the cryofuge into a replica of the microstructured electric trap has allowed us to perform a first systematic study of collisions between cold methyl fluoride molecules. We measure loss rate coefficients up to 4×10-8cm3/s for molecules at a temperature of about 350mK. Moreover, the tunable homogeneous electric fields inside our trap allow us to explore the effect of electric fields on the collision rate. We observe a strong electric field dependence, with the collision rate decreasing by a factor of 2 when increasing the offset field strength from 0.5kV/cm to 2.4kV/cm. We perform theoretical calculations of the collision rate which closely reproduce the relative electric field dependence, demonstrating that the losses are primarily due to dipolar relaxation. The ability to tune losses is promising for observing thermalization between molecules due to elastic collisions.
M. Koller et al., Phys. Rev. Lett. 128, 203401 (2022)
Interactions between polar molecules and Rydberg atoms
A new approach for detecting molecules based on Rydberg atoms
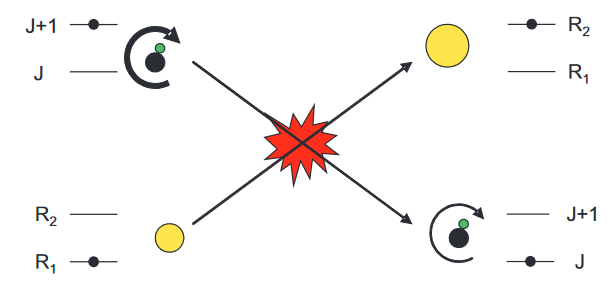
Efficient detection of molecules has been a major bottleneck in our experiments. For the most part, we have relied on quadrupole mass spectrometers for this purpose. While quadrupole mass spectrometers are a robust, molecule species independent, commercially available detection technique, this comes at the expense of a very low detection efficiency. We generally detect only about one in every 10000 molecules, considerably limiting our experiments.
As an alternative means of detecting molecules, we have proposed the use of Rydberg atoms, i.e. atoms with a single electron excited to very high lying states, orbiting at a large distance from the rest of the atom. A first key realization was that a single Rydberg atom would be able to sense the electric field from a single polar molecules at distances of 1μm and more. A second key realisation was that the presence of a single molecule can be imprinted on a Rydberg atom when they simply fly past each other via Förster resonant energy transfer (FRET). In FRET, a rotational excitation in the molecule is transferred to the Rydberg atom (or vice versa) via resonant dipole-dipole interactions. Basic calculations show that this process occurs with extremely high cross sections, allowing for efficient and moreover nondestructive detection of molecules.
M. Zeppenfeld, EPL 118, 13002 (2017)
Investigation of molecule-Rydberg-atom interactions at room temperature
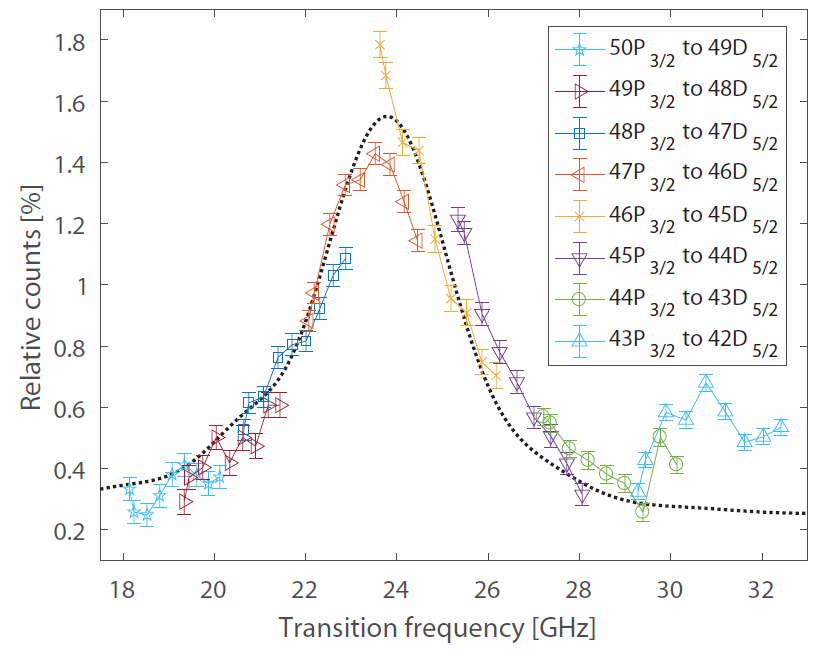
As a first experiment, we have investigated interactions between ammonia molecules and rubidium Rydberg atoms at room temperature. Rubidium atoms in a thermal vapor are excited to Rydberg states via single photon excitation by a pulsed dye laser between a pair of capacitor plates. Subsequently, interactions with a low pressure gas of ammonia molecules injected into the same vacuum chamber can take place for up to about 10μs. Finally, the Rydberg atoms are field ionized via a voltage ramp applied to the capacitor plates, and the resulting electrons are extracted via a mesh in one of the capacitor plates and detected with a channel electron multiplier. The electric field strength at which ionization takes place allows to infer the internal state of the Rydberg atom prior to ionization.
Exciting rubidium atoms to an nP state with n ≈ 46 allows Förster resonant energy transfer to take place with the inversion transition in ammonia. Our setup allows this interaction to be studies in detail, including ammonia pressure dependence, dependence on the initial and final Rydberg state, and electric field dependence. The dependence on the electric field strength is particularly interesting since tuning the electric field strongly shifts the separation between Rydberg states, thereby affecting the resonance condition for interactions with ammonia molecules. We observe a strong dependence of the interactions on the electric field strength. In combination with varying the initial Rydberg state, changing the electric field allows the Rydberg transition to be tuned across the ammonia inversion transition, allowing to record a low resolution spectrum of this transition.
F. Jarisch et al., New J. Phys. 20, 113044 (2018)
Precision Spectroscopy of polar molecules using Rydberg atoms
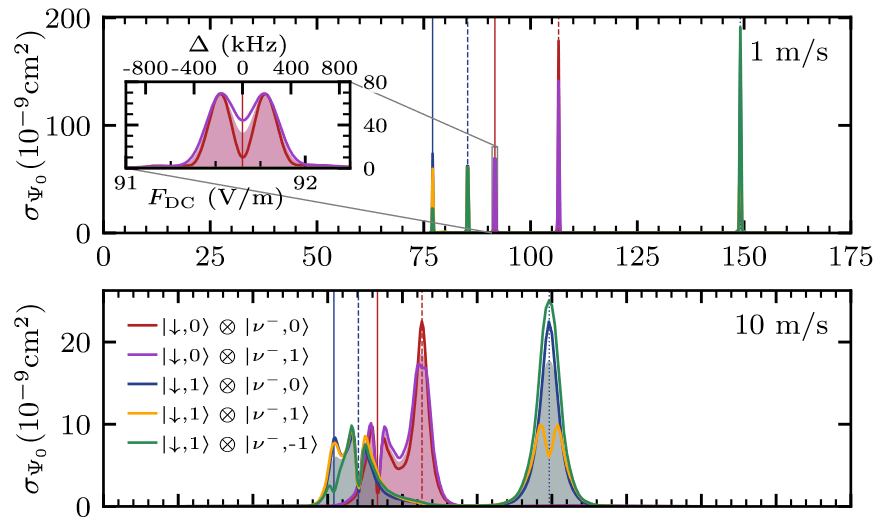
We have performed detailed theoretical calculations of the molecule-Rydberg-atom interaction cross sections, based on position dependent dipole-dipole interactions, and including the full set of relevant internal states for the molecules and Rydberg atoms. For a relative velocity between an ammonia molecule and a rubidium Rydberg atom of 1m/s, interaction cross sections exceed 10-7cm2. Moreover, at such low velocities we calculate extremely narrow features as a function of the electric field strength, due to the interaction resonance condition. Our work demonstrates the possibility to perform a new type of precision molecular spectroscopy, with photons in traditional spectroscopy being replaced by Rydberg atoms.
S. Patsch et al., J. Phys. Chem. Lett. 13, 10728 (2022)
Nondestructive detection of a slow molecular beam via Rydberg atoms
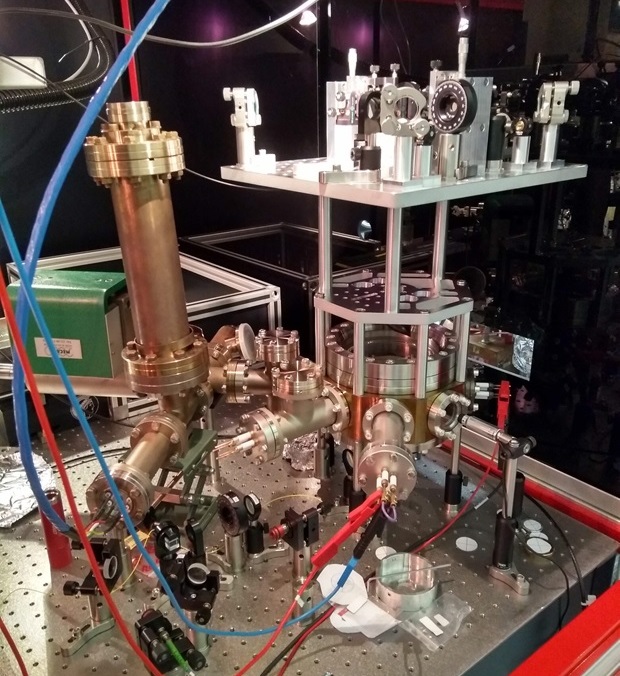
We have experimentally demonstrated the ability to nondestructively detect a slow beam of molecules produced via velocity filtered using Rydberg atoms. Rubidium atoms are loaded into a magneto optical trap located directly behind the end of the quadrupole guide used to transversely confine the slow molecular beam. Rubidium atoms are excited to Rydberg states using two photon excitation, and are subsequently allowed to interact with the slow molecules for about 100μs. The Rydberg atoms are detected state selectively using field ionization.
The Rydberg-based molecule detection allows to characterize our source of slow molecules. We measure the signal dependence on the molecule nozzle backing pressure and on the high voltages applied to the quadrupole guide electrodes. Moreover, a 2kHz shot rate of the experiment allows to perform time of flight measurements on the molecules and extract a velocity distribution for the slow molecular beam. Our results suggest that further improvements to the experiment would allow to detect slow molecule with an efficiency exceeding that of a commercial quadrupole mass spectrometer.
High order modes of optical resonators
A mathematical framework to describe electromagnetic beams using spheroidal coordinates
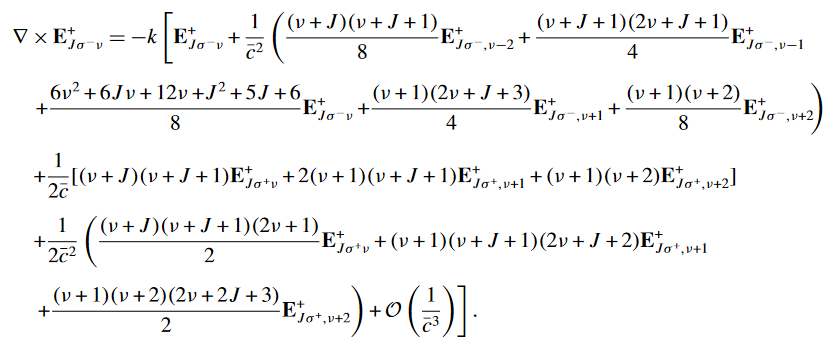
In optics, the paraxial approximation is a powerful tool to obtain approximate solutions to Maxwell's equations which describe beams of electromagnetic radiation. We show that the Gauss-Laugerre solutions to the paraxial approximation naturally arise from exact solutions to the wave equation in the short wavelength limit when the wave equation is separated and solved in oblate spheroidal coordinates. In particular, while it has long been known that the angle functions for the solutions in spheroidal coordinates match the dependence of solutions in the paraxial approximation transverse to the beam direction, we show that the same correspondence exists between the radial functions and the dependence of the solutions in the paraxial approximation along the beam axis.
Examining the geometry of spheroidal coordinates provides a natural explanation for the geometry of paraxial beams. Our results provide a natural approach to calculating corrections to the paraxial approximation.
M. Zeppenfeld, New J. Phys. 11, 073007 (2009)
Using spheroidal wave functions to calculate the fine structure of an optical resonator
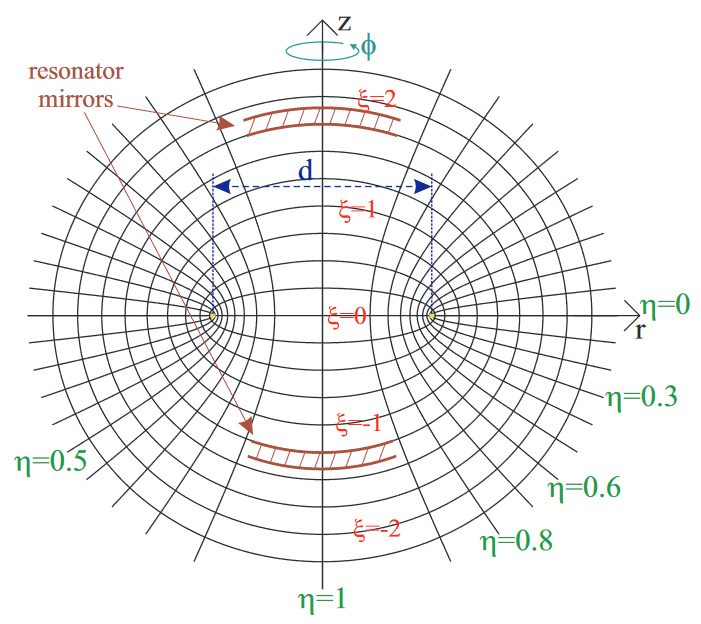
Using spheroidal wave functions allows us to calculate the eigenmodes of an optical Fabry-Perot resonator with a precision beyond what is possible using the paraxial approximation. Whereas the paraxial approximation predicts sets of resonator modes which are degenerate, our calculation shows that this degeneracy is lifted, with the precise frequency of resonator modes depending on the orbital angular momentum of light. Moreover, we show that the resonator frequencies depend on a coupling between the orbital angular momentum and spin angular momentum of light. Our results are relevant in a variety of settings, including microresonators, high-finesse resonators, and microwave resonators.
M. Zeppenfeld et al., Opt. Expr. 18, 9580 (2010)
Observing resonator fine structure in a high-finesse optical resonator
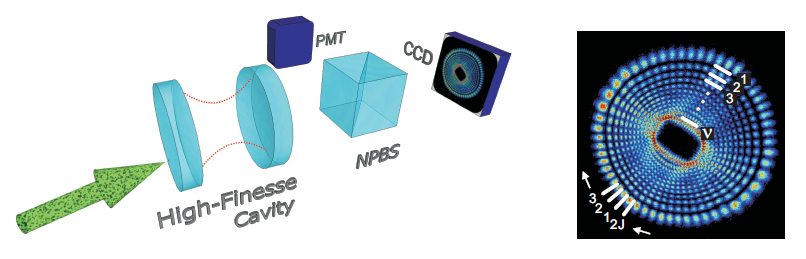
Using a high-finesse resonator, we observe resonator fine structure and spin-orbit coupling. High-order resonator modes are split according to their orbital angular momentum, resulting in mode profiles corresponding to Laguerre-Gaussian modes. Moreover, coupling light of opposite circular polarisation into the resonator results in observation of two distinct series of resonator modes, demonstrating spin-orbit coupling. Our results represent the first observation of these effects in the optical domain.